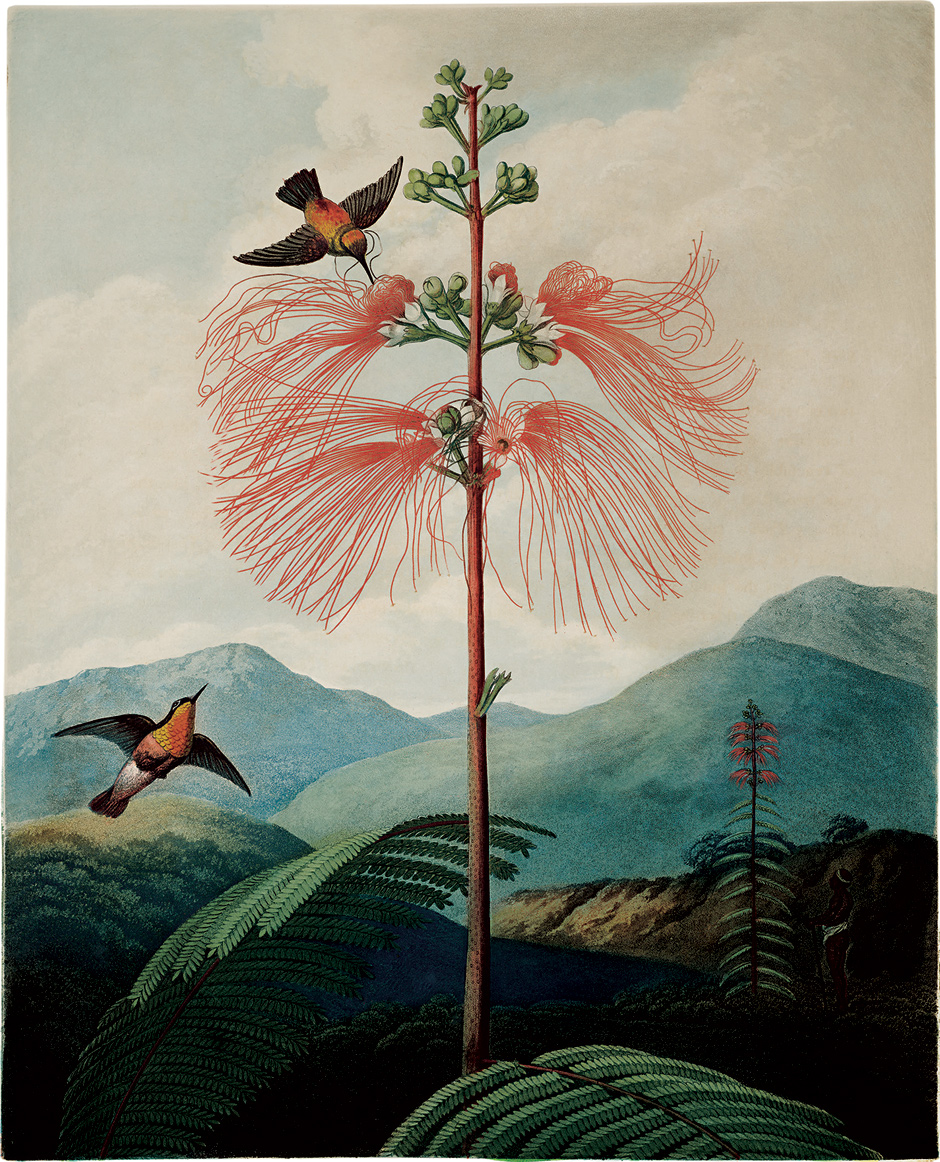
Charles Darwin’s last book, published in 1881, was a study of the humble earthworm. His main theme—expressed in the title, The Formation of Vegetable Mould through the Action of Worms—was the immense power of worms, in vast numbers and over millions of years, to till the soil and change the face of the earth. But his opening chapters are devoted more simply to the “habits” of worms.
Worms can distinguish between light and dark, and they generally stay underground, safe from predators, during daylight hours. They have no ears, but if they are deaf to aerial vibration, they are exceedingly sensitive to vibrations conducted through the earth, as might be generated by the footsteps of approaching animals. All of these sensations, Darwin noted, are transmitted to collections of nerve cells (he called them “the cerebral ganglia”) in the worm’s head.
“When a worm is suddenly illuminated,” Darwin wrote, it “dashes like a rabbit into its burrow.” He noted that he was “at first led to look at the action as a reflex one,” but then observed that this behavior could be modified—for instance, when a worm was otherwise engaged, it showed no withdrawal with sudden exposure to light.
For Darwin, the ability to modulate responses indicated “the presence of a mind of some kind.” He also wrote of the “mental qualities” of worms in relation to their plugging up their burrows, noting that “if worms are able to judge…having drawn an object close to the mouths of their burrows, how best to drag it in, they must acquire some notion of its general shape.” This moved him to argue that worms “deserve to be called intelligent, for they then act in nearly the same manner as a man under similar circumstances.”
As a boy, I played with the earthworms in our garden (and later used them in research projects), but my true love was for the seashore, and especially tidal pools, for we nearly always took our summer holidays at the seaside. This early, lyrical feeling for the beauty of simple sea creatures became more scientific under the influence of a biology teacher at school and our annual visits with him to the Marine Station at Millport in southwest Scotland, where we could investigate the immense range of invertebrate animals on the seashores of Cumbrae. I was so excited by these Millport visits that I thought I would like to become a marine biologist myself.
If Darwin’s book on earthworms was a favorite of mine, so too was George John Romanes’s 1885 book Jelly-Fish, Star-Fish, and Sea-Urchins: Being a Research on Primitive Nervous Systems, with its simple, fascinating experiments and beautiful illustrations. For Romanes, Darwin’s young friend and student, the seashore and its fauna were to be passionate and lifelong interests, and his aim above all was to investigate what he regarded as the behavioral manifestations of “mind” in these creatures.
I was charmed by Romanes’s personal style. (His studies of invertebrate minds and nervous systems were most happily pursued, he wrote, in “a laboratory set up upon the sea-beach…a neat little wooden workshop thrown open to the sea-breezes.”) But it was clear that correlating the neural and the behavioral was at the heart of Romanes’s enterprise. He spoke of his work as “comparative psychology,” and saw it as analogous to comparative anatomy.
Louis Agassiz had shown, as early as 1850, that the jellyfish Bougainvillea had a substantial nervous system, and by 1883 Romanes demonstrated its individual nerve cells (there are about a thousand). By simple experiments—cutting certain nerves, making incisions in the bell, or looking at isolated slices of tissue—he showed that jellyfish employed both autonomous, local mechanisms (dependent on nerve “nets”) and centrally coordinated activities through the circular “brain” that ran along the margins of the bell.
By 1883, Romanes was able to include drawings of individual nerve cells and clusters of nerve cells, or ganglia, in his book Mental Evolution in Animals. “Throughout the animal kingdom,” Romanes wrote,
nerve tissue is invariably present in all species whose zoological position is not below that of the Hydrozoa. The lowest animals in which it has hitherto been detected are the Medusae, or jelly-fishes, and from them upwards its occurrence is, as I have said, invariable. Wherever it does occur its fundamental structure is very much the same, so that whether we meet with nerve-tissue in a jelly-fish, an oyster, an insect, a bird, or a man, we have no difficulty in recognizing its structural units as everywhere more or less similar.
At the same time that Romanes was vivisecting jellyfish and starfish in his seaside laboratory, the young Sigmund Freud, already a passionate Darwinian, was working in the lab of Ernst Brücke, a physiologist in Vienna. His special concern was to compare the nerve cells of vertebrates and invertebrates, in particular those of a very primitive vertebrate (Petromyzon, a lamprey) with those of an invertebrate (a crayfish). While it was widely held at the time that the nerve elements in invertebrate nervous systems were radically different from those of vertebrate ones, Freud was able to show and illustrate, in meticulous, beautiful drawings, that the nerve cells in crayfish were basically similar to those of lampreys—or human beings.
Advertisement
And he grasped, as no one had before, that the nerve cell body and its processes—dendrites and axons—constituted the basic building blocks and the signaling units of the nervous system. Eric Kandel, in his book In Search of Memory: The Emergence of a New Science of Mind (2006), speculates that if Freud had stayed in basic research instead of going into medicine, perhaps he would be known today as “a co-founder of the neuron doctrine, instead of as the father of psychoanalysis.”
Although neurons may differ in shape and size, they are essentially the same from the most primitive animal life to the most advanced. It is their number and organization that differ: we have a hundred billion nerve cells, while a jellyfish has a thousand. But their status as cells capable of rapid and repetitive firing is essentially the same.
The crucial role of synapses—the junctions between neurons where nerve impulses can be modulated, giving organisms flexibility and a whole range of behaviors—was clarified only at the close of the nineteenth century by the great Spanish anatomist Santiago Ramón y Cajal, who looked at the nervous systems of many vertebrates and invertebrates, and by C.S. Sherrington in England (it was Sherrington who coined the word “synapse” and showed that synapses could be excitatory or inhibitory in function).
In the 1880s, however, despite Agassiz’s and Romanes’s work, there was still a general feeling that jellyfish were little more than passively floating masses of tentacles ready to sting and ingest whatever came their way, little more than a sort of floating marine sundew.
But jellyfish are hardly passive. They pulsate rhythmically, contracting every part of their bell simultaneously, and this requires a central pacemaker system that sets off each pulse. Jellyfish can change direction and depth, and many have a “fishing” behavior that involves turning upside down for a minute, spreading their tentacles like a net, and then righting themselves, which they do by virtue of eight gravity-sensing balance organs. (If these are removed, the jellyfish is disoriented and can no longer control its position in the water.) If bitten by a fish, or otherwise threatened, jellyfish have an escape strategy—a series of rapid, powerful pulsations of the bell—that shoots them out of harm’s way; special, oversized (and therefore rapidly responding) neurons are activated at such times.
Of special interest and infamous reputation among divers is the box jellyfish (Cubomedusae)—one of the most primitive animals to have fully developed image-forming eyes, not so different from our own. The biologist Tim Flannery, in an article in these pages, writes of box jellyfish:
They are active hunters of medium-sized fish and crustaceans, and can move at up to twenty-one feet per minute. They are also the only jellyfish with eyes that are quite sophisticated, containing retinas, corneas, and lenses. And they have brains, which are capable of learning, memory, and guiding complex behaviors.1
We and all higher animals are bilaterally symmetrical, have a front end (a head) containing a brain, and a preferred direction of movement (forward). The jellyfish nervous system, like the animal itself, is radially symmetrical and may seem less sophisticated than a mammalian brain, but it has every right to be considered a brain, generating, as it does, complex adaptive behaviors and coordinating all the animal’s sensory and motor mechanisms. Whether we can speak of a “mind” here (as Darwin does in regard to earthworms) depends on how one defines “mind.”
We all distinguish between plants and animals. We understand that plants, in general, are immobile, rooted in the ground; they spread their green leaves to the heavens and feed on sunlight and soil. We understand that animals, in contrast, are mobile, moving from place to place, foraging or hunting for food; they have easily recognized behaviors of various sorts. Plants and animals have evolved along two profoundly different paths (fungi have yet another), and they are wholly different in their forms and modes of life.
And yet, Darwin insisted, they were closer than one might think. He wrote a series of botanical books, culminating in The Power of Movement in Plants (1880), just before his book on earthworms. He thought the powers of movement, and especially of detecting and catching prey, in the insectivorous plants so remarkable that, in a letter to the botanist Asa Gray, he referred to Drosera, the sundew, only half-jokingly as not only a wonderful plant but “a most sagacious animal.”
Advertisement
Darwin was reinforced in this notion by the demonstration that insect-eating plants made use of electrical currents to move, just as animals did—that there was “plant electricity” as well as “animal electricity.” But “plant electricity” moves slowly, roughly an inch a second, as one can see by watching the leaflets of the sensitive plant (Mimosa pudica) closing one by one along a leaf that is touched. “Animal electricity,” conducted by nerves, moves roughly a thousand times faster.2
Signaling between cells depends on electrochemical changes, the flow of electrically charged atoms (ions), in and out of cells via special, highly selective molecular pores or “channels.” These ion flows cause electrical currents, impulses—action potentials—that are transmitted (directly or indirectly) from one cell to another, in both plants and animals.
Plants depend largely on calcium ion channels, which suit their relatively slow lives perfectly. As Daniel Chamovitz argues in his book What a Plant Knows (2012), plants are capable of registering what we would call sights, sounds, tactile signals, and much more. Plants know what to do, and they “remember.” But without neurons, plants do not learn in the same way that animals do; instead they rely on a vast arsenal of different chemicals and what Darwin termed “devices.” The blueprints for these must all be encoded in the plant’s genome, and indeed plant genomes are often larger than our own.
The calcium ion channels that plants rely on do not support rapid or repetitive signaling between cells; once a plant action potential is generated, it cannot be repeated at a fast enough rate to allow, for example, the speed with which a worm “dashes…into its burrow.” Speed requires ions and ion channels that can open and close in a matter of milliseconds, allowing hundreds of action potentials to be generated in a second. The magic ions, here, are sodium and potassium ions, which enabled the development of rapidly reacting muscle cells, nerve cells, and neuromodulation at synapses. These made possible organisms that could learn, profit by experience, judge, act, and finally think.
This new form of life—animal life—emerging perhaps 600 million years ago conferred great advantages, and transformed populations rapidly. In the so-called Cambrian explosion (datable with remarkable precision to 542 million years ago), a dozen or more new phyla, each with very different body plans, arose within the space of a million years or less—a geological eye-blink. The once peaceful pre-Cambrian seas were transformed into a jungle of hunters and hunted, newly mobile. And while some animals (like sponges) lost their nerve cells and regressed to a vegetative life, others, especially predators, evolved increasingly sophisticated sense organs, memories, and minds.
It is fascinating to think of Darwin, Romanes, and other biologists of their time searching for “mind,” “mental processes,” “intelligence,” even “consciousness” in primitive animals like jellyfish, and even in protozoa. A few decades afterward, radical behaviorism would come to dominate the scene, denying reality to what was not objectively demonstrable, denying in particular any inner processes between stimulus and response, deeming these as irrelevant or at least beyond the reach of scientific study.
Such a restriction or reduction indeed facilitated studies of stimulation and response, both with and without “conditioning,” and it was Pavlov’s famous studies of dogs that formalized—as “sensitization” and “habituation”—what Darwin had observed in his worms.3
As Konrad Lorenz wrote in The Foundations of Ethology, “an earthworm [that] has just avoided being eaten by a blackbird…is indeed well-advised to respond with a considerably lowered threshold to similar stimuli, because it is almost certain that the bird will still be nearby for the next few seconds.” This lowering of threshold, or sensitization, is an elementary form of learning, even though it is nonassociative and relatively short-lived. Correspondingly, a diminution of response, or habituation, occurs when there is a repeated but insignificant stimulus—something to be ignored.
It was shown within a few years of Darwin’s death that even single-cell organisms like protozoa could exhibit a range of adaptive responses. In particular, Herbert Spencer Jennings showed that the tiny, stalked, trumpet-shaped unicellular organism Stentor employs a repertoire of at least five different responses to being touched, before finally detaching itself to find a new site if these basic responses are ineffective. But if it is touched again, it will skip the intermediate steps and immediately take off for another site. It has become sensitized to noxious stimuli, or, to use more familiar terms, it “remembers” its unpleasant experience and has learned from it (though the memory lasts only a few minutes). If, conversely, Stentor is exposed to a series of very gentle touches, it soon ceases to respond to these at all—it has habituated.
Jennings described his work with sensitization and habituation in organisms like Paramecium and Stentor in his 1906 book Behavior of the Lower Organisms. Although he was careful to avoid any subjective, mentalistic language in his description of protozoan behaviors, he did include an astonishing chapter at the end of his book on the relation of observable behavior to “mind.”
He felt that we humans are reluctant to attribute any qualities of mind to protozoa because they are so small:
The writer is thoroughly convinced, after long study of the behaviour of this organism, that if Amoeba were a large animal, so as to come in the everyday experience of human beings, its behaviour would at once call forth the attribution to it of states of pleasure and pain, of hunger, desire, and the like, on precisely the same basis as we attribute these things to the dog.
Jennings’s vision of a highly sensitive, dog-size Amoeba is almost cartoonishly the opposite of Descartes’s notion of dogs as so devoid of feelings that one could vivisect them without compunction, taking their cries as purely “reflex” reactions of a quasi-mechanical kind.
Sensitization and habituation are crucial for the survival of all living organisms. These elementary forms of learning are short-lived—a few minutes at most—in protozoa and plants; longer-lived forms require a nervous system.
While behavioral studies flourished, there was almost no attention paid to the cellular basis of behavior—the exact role of nerve cells and their synapses. Investigations in mammals—involving, for example, the hippocampal or memory systems in rats—presented almost insuperable technical difficulties, due to the tiny size and extreme density of neurons (there were difficulties, moreover, even if one could record electrical activity from a single cell, in keeping it alive and fully functioning for the duration of protracted experiments).
Faced with such difficulties in his anatomical studies in the early twentieth century, Ramón y Cajal—the first and greatest microanatomist of the nervous system—had turned to study simpler systems: those of young or fetal animals, and those of invertebrates (insects, crustaceans, cephalopods, etc.). For similar reasons, Eric Kandel, when he embarked in the 1960s on a study of the cellular basis of memory and learning, sought an animal with a simpler and more accessible nervous system. He settled on the giant sea snail Aplysia, which has 20,000 or so neurons, distributed in ten or so ganglia of about 2,000 neurons apiece. It also has particularly large neurons—some even visible to the naked eye—connected with one another in fixed anatomical circuits.
That Aplysia might be considered too low a form of life for studies of memory did not discountenance Kandel, despite some skepticism from his colleagues—any more than it had discountenanced Darwin when he spoke of the “mental qualities” of earthworms. “I was beginning to think like a biologist,” Kandel writes, recalling his decision to work with Aplysia. “I appreciated that all animals have some form of mental life that reflects the architecture of their nervous system.”
As Darwin had looked at an escape reflex in worms and how it might be facilitated or inhibited in different circumstances, Kandel looked at a protective reflex in Aplysia, the withdrawal of its exposed gill to safety, and the modulation of this response. Recording from (and sometimes stimulating) the nerve cells and synapses in the abdominal ganglion that governed these responses, he was able to show that relatively short-term memory and learning—as involved in habituation and sensitization—depended on functional changes in synapses; but longer-term memory, which might last several months, went with structural changes in the synapses. (In neither case was there any change in the actual circuits.)
As new technologies and concepts emerged in the 1970s, Kandel and his colleagues were able to complement these electrophysiological studies of memory and learning with chemical ones: “We wanted to penetrate the molecular biology of a mental process, to know exactly what molecules are responsible for short-term memory.” This entailed, in particular, studies of the ion channels and neurotransmitters involved in synaptic functions—monumental work that earned Kandel a Nobel Prize.
Where Aplysia has only 20,000 neurons distributed in ganglia throughout its body, an insect may have up to a million nerve cells, all concentrated in one brain, and despite its tiny size may be capable of extraordinary cognitive feats. Thus bees are expert in recognizing different colors, smells, and geometric shapes presented in a laboratory setting, as well as systematic transformations of these. And of course, they show superb expertise in the wild or in our gardens, where they recognize not only the patterns and smells and colors of flowers, but can remember their locations and communicate these to their fellow bees.
It has even been shown, in a highly social species of paper wasp, that individuals can learn and recognize the faces of other wasps. Such face learning has hitherto been described only in mammals; it is fascinating that a cognitive power so specific can be present in insects as well.
We often think of insects as tiny automata—robots with everything built-in and programmed. But it is increasingly evident that insects can remember, learn, think, and communicate in quite rich and unexpected ways. Much of this, doubtless, is built-in—but much, too, seems to depend on individual experience.
Whatever the case with insects, there is an altogether different situation with those geniuses among invertebrates, the cephalopods, consisting of octopuses, cuttlefish, and squid. Here, as a start, the nervous system is much larger—an octopus may have half a billion nerve cells distributed between its brain and its “arms” (a mouse, by comparison, has only 75 to 100 million). There is a remarkable degree of organization in the octopus brain, with dozens of functionally distinct lobes in the brain and similarities to the learning and memory systems of mammals.
Cephalopods are not only easily trained to discriminate test shapes and objects, but some reportedly can learn by observation, a power otherwise confined to certain birds and mammals. They have remarkable powers of camouflage, and can signal complex emotions and intentions by changing their skin colors, patterns and textures.
Darwin noted in The Voyage of the Beagle how an octopus in a tidal pool seemed to interact with him, by turns watchful, curious, and even playful. Octopuses can be domesticated to some extent, and their keepers often empathize with them, feeling some sense of mental and emotional proximity. Whether one can use the “C” word—consciousness—in regard to cephalopods can be argued all ways. But if one allows that a dog may have consciousness of an individual and significant sort, one has to allow it for an octopus, too.
Nature has employed at least two very different ways of making a brain—indeed, there are almost as many ways as there are phyla in the animal kingdom. Mind, to varying degrees, has arisen or is embodied in all of these, despite the profound biological gulf that separates them from one other, and us from them.4
This Issue
April 24, 2014
The Future of Europe
‘The Smallest Possible Disaster’
-
1
“They’re Taking Over!,” The New York Review, September 26, 2013. ↩
-
2
In 1859, Hermann von Helmholtz was able to measure the speed of nerve conduction at 80 feet per second. If we speed up a time-lapse film of plant movement by a thousandfold, plant behaviors start to look animal-like and may even appear “intentional.” ↩
-
3
Pavlov used dogs in his famous experiments on “conditioned reflexes,” and the conditioning stimulus was usually a bell, which the dogs learned to associate with food. But on one occasion, in 1924, there was a huge flood in the laboratory that nearly drowned the dogs. After this, many of the dogs were sensitized, even terrified, by the sight of water for the rest of their lives. Extreme or long-lasting sensitization underlies PTSD, in dogs as in humans. ↩
-
4
I would like to thank several colleagues for discussions that have informed this article (though mistakes, of course, are mine alone)—especially Susan R. Barry, Ralph Greenspan, Roger Hanlon, Eric Kandel, and Diana Reiss. ↩